Khani L, Taghizade Salari M, Gholami K, Ahmadnia H, Hasanpour M, Iranshahy M et al . Investigating the Effect of Administration of Urolithin A and B on Learning and Memory İmpairments and Anxiety-like Behaviors Caused by Alzheimer’s İnduced by Intracerebroventricular İnjection of Streptozotocin in Male Wistar Rat. Intern Med Today 2023; 29 (2) :87-97
URL:
http://imtj.gmu.ac.ir/article-1-3998-en.html
1- Department of Biology, Faculty of Science, Ferdowsi University of Mashhad, Mashhad, Iran.
2- Biotechnology Research Center, Pharmaceutical Technology Institute, Mashhad University of Medical Sciences, Mashhad, Iran.
3- Department of Pharmacognosy, School of Pharmacy, Mashhad University of Medical Sciences, Mashhad, Iran.
4- Department of Biology, Faculty of Science, Ferdowsi University of Mashhad, Mashhad, Iran. , behnam@um.ac.ir
Full-Text [PDF 714 kb]
(337 Downloads)
|
Abstract (HTML) (1329 Views)
Full-Text: (215 Views)
Alzheimer’s disease is one of the most common neurodegenerative dementia diseases [1]. Dementia syndrome is characterized by various symptoms, such as problems with memory, speech, problem-solving, and other cognitive skills, that affect a person's ability to perform daily activities. These problems occur due to the damage to or destruction of the nerve cells in the brain regions that are involved in cognitive function. Studies show that the prevalence of Alzheimer’s disease grows steadily with increasing age and imposes a lot of financial and psychological costs on societies [2].
Pathophysiological changes in this disease include beta-amyloid accumulation, the creation of neurofibrillary tangles, and neurodegeneration resulting from uncontrolled activation of microglia, leading to the release of neurotoxins and inflammatory agents. Individuals with such changes may be asymptomatic or may exhibit clinical symptoms ranging from memory deficits to severe and disabling loss of memory and cognitive function. As the disease progresses, additional symptoms may manifest, including confusion, disorientation, mood changes, aggression, and eventually hallucinations [3]. In 2020, there were approximately 50 million Alzheimer’s patients worldwide, and this number is predicted to double every 5 years, reaching 152 million by 2050 [4].
Currently, only four drugs are approved by the Food and Drug Administration to treat Alzheimer’s. Three of these medications are acetylcholinesterase inhibitors (donepezil, galantamine, and rivastigmine), and the other one is an N-methyl-D-aspartate (NMDA) receptor antagonist (memantine). However, these drugs help to improve the symptoms to a limited extent as they fail to prevent brain atrophy, the loss of neurons, and as a result, the gradual deterioration of cognitive function. Therefore, the development of disease-modifying therapies to control the progression of Alzheimer’s disease has taken priority throughout the world [5]. Consequently, this disease has become one of the significant challenges in the field of medical care in the present century. Despite all the research efforts, no accurate and effective treatment option has been provided for this disease, and research is still ongoing to find a suitable and compatible treatment [6].
Difficulty in forming and maintaining new event memories is usually the first and most prominent clinical symptom of Alzheimer’s disease [7]. Learning and memory in Alzheimer’s disease are affected due to the gradual decline of cognitive abilities, impairing short and long-term memory early in the course of the disease [8]. The presence of amyloid plaques and neurofibrillary tangles causes neurodegeneration, and the extent of these neurological changes in the cortex and hippocampus, which are key areas related to learning and memory, is associated with cognitive deficits in Alzheimer’s disease [9].
Anxiety is the third most common symptom of Alzheimer’s disease, with a prevalence of about 40%. Anxiety can be especially present among patients with mild cognitive impairment, mild dementia, or early-stage Alzheimer’s disease and can cause progression or conversion to clinical Alzheimer’s syndrome [10]. From a pathological perspective, anxiety is considered equivalent to natural fear and manifests with abnormal activities and disorders in mood, thinking, and behavior [11]. Several interconnected neural circuits, including the hippocampus, prefrontal cortex, and amygdala, are involved in this mental disorder [12].
According to researchers, anxiety behaviors present in several stages. In the pre-dementia stage, the patient shows very mild cognitive impairment, but can independently do his daily tasks. In the mild dementia stage, impairment in learning and memory are evident as clinical features in most patients. In some affected people, there can be aphasia and vision defects, partial indifference, and also mild depression. In the stage of moderate dementia, in addition to severe memory and cognitive function disorders, the patient may lose his emotional control. At this stage, affected individuals face significant difficulties in logical reasoning, planning, organization, reading, and writing. In the severe dementia stage, the patient's memory and cognitive function are seriously affected. Aggression, movement disorders, excessive fatigue, and indifference to the surrounding environment are also observed [13].
Three methods are adopted to induce experimental Alzheimer’s disease in mouse models, which include intraventricular-cerebral injection of streptozotocin, bilateral carotid artery occlusion, and inhibition of brain mitochondrial cytochrome oxidase by sodium azide. In rats, intraventricular-cerebral injection of streptozotocin disrupts insulin signal transmission pathways in the brain by desensitizing the insulin receptors of nerve cells [14]. This substance, in a dose-dependent and progressive manner, leads to the development of pathologies similar to Alzheimer’s disease, including brain glucose metabolism disorders, decreased brain insulin resistance, deposition of amyloid plaques, tau protein hyperphosphorylation, synaptic dysfunction, oxidative stress, and neuronal destruction [15].
Flavonoids are the largest group of phenolic compounds that are widely present in plants and are part of the diet [16]. These secondary metabolites exhibit a range of biological effects, including anti-inflammatory, antioxidant [17], antibacterial, antiviral [18], anti-allergic, and anti-cancer [19]. Ellagitannins, as one of the main groups of hydrolyzable tannins, are a group of flavonoids found in berries, including strawberries, raspberries, and blackberries, as well as in pomegranates, tea, walnuts, and grapes [20, 21]. Foods rich in ellagitannins are a good source of antioxidant polyphenols that have beneficial effects on health, including free radical scavenging [22]. Ellagitannins are hydrolyzed in the intestine and lactonized to ellagic acid. Although both of these substances are poorly absorbed in the intestine, they are catabolized in the end part of the digestive tract by the intestinal microbiota to urolithin, which has a higher absorption rate and better biocompatibility [23].
The concentration of urolithins in plasma, urine, and feces varies significantly from individual to individual, which is probably due to the presence of different microbial flora in the gut microbiota. Urolithins are of increasing interest due to various biological activities, such as cardiovascular protection, anti-inflammatory activity, anti-cancer properties, anti-diabetic activity, and anti-aging and antimicrobial properties [24]. The main isomers found in human biological fluids and tissues after consuming ellagitannin-rich foods are urolithin A and urolithin B [25], which reach plasma and systemic tissues within 3 to 4 days after consumption, exert their biological function, and finally, enter the liver and are excreted through urine [26].
Considering the antioxidant and anti-inflammatory effects of urolithin compounds (urolithin A and B) and their neuroprotective role in brain disorders, especially memory [12], the present study aimed to investigate the protective effects of urolithin A and B on improving learning and memory impairments as well as anxiety-like behaviors following the induction of Alzheimer’s disease in male Wistar rats through intraventricular-cerebral injection of streptozotocin.
Materials and Methods
Animals and studied groups
The statistical population consisted of 70 adult male Wistar rats weighing 250 g. These rats were kept in laboratory conditions with a 12-hour light/dark cycle and a temperature of 24°C, and they had access to sufficient food and water during the experiment. In order to habituate the rats to the environment, the experiments were initiated one week after their transfer to the animal facility. All experiments were carried out according to the code of ethics of the Bioethics Committee of Ferdowsi University of Mashhad (IR.UM.REC.1402.058).
The rats were randomly divided into 10 groups of seven as follows: 1) healthy control group, 2) sham group: streptozotocin solvent (0.1% ascorbic acid in saline) injected intracerebroventricularly and urolithin drug solvent (dimethyl sulfoxide [DMSO]) injected intraperitoneally, 3) positive control group (memantine): injected 3 mg/kg body weight of streptozotocin intracerebroventricularly and 100 µl of memantine with a concentration of 0.5 mg/kg body weight intraperitoneally, 4) negative control group (experimental Alzheimer’s): injected 3 mg/kg body weight of streptozotocin intracerebroventricularly, 5) treatment groups 1 to 3: after the induction of Alzheimer’s disease with intraventricular-cerebral injection of 3 mg/kg of body weight of streptozotocin, intraperitoneally injected urolitin A with a concentration of 10, 20, and 40 mg/kg of body weight, respectively, and 6) treatment groups 4 to 6: after the induction of Alzheimer’s disease with an intraventricular-cerebral injection of 3 mg/kg of streptozotocin, intraperitoneally injected urolitin B with a concentration of 5, 10, and 20 mg/kg of body weight, intraperitoneally.
For the purpose of intraventricular-cerebral injection, initially, stereotaxic surgery and lateral intraventricular cannulation (right side) were performed, and then the injection was administered. Drug treatments in the memantine group, experimental Alzheimer’s group, and treatment groups 1 to 6 were performed one day after streptozotocin injection daily for 2 weeks.
Stereotaxic surgery
Stereotaxic device consists of three calipers in three spatial axes. Ear bars are two pointed bars that are placed inside the animal's ears and should be the same size on both sides. The middle bar, in which the animal's front tooth is placed, is set at 3.3 mm for rats. There is also a section for the guide cannula which is placed on the vertical bar. The rat's head is fixed in such a way that the skull becomes upright and straight.
The animals were anesthetized by intraperitoneal injection of ketamine and xylazine solution (5:2 mg/kg). Upon cutting the hair of the head, the rats were fixed in the stereotaxic device. The skin of the head was cut by a scalpel from the eyes to the ears at a one-centimeter length. A few drops of epinephrine were administered in the area to stop the bleeding. Following that, the skull surface was cleaned with an alcohol swab, and bregma and lambda were determined based on the Paxinos Watson rat brain atlas [27].
A 0.5 mm drill created a hole in the animal's skull 0.8 mm from the bregma, 1.4 mm from the middle groove towards the right lateral ventricle, and 3.6 mm deep [28]. Following the insertion of the guide cannula into the desired area, dental cement was poured around the cannula to keep it fixed. After the surgery, streptozotocin at a dose of 3 μg in 10 μl of 1.0% ascorbic acid-saline solution was injected at a rate of 5.0 μl/min into the lateral ventricle of the negative control group and six treatment groups via a Hamilton syringe connected to a 27-gauge cannula. The Sham group also received streptozotocin solution (1.0% ascorbic acid in saline) post-surgery using a Hamilton syringe via a stereotaxic device.
Behavioral tests
Passive preventive learning test
The shuttle box test is a validated experimental method used for the short-term assessment of learning and memory. This box consists of two separate dark and light parts. The light part has a 5-watt lamp, and the bottom of the dark part has stainless-steel rods at 1-centimeter distances. The dark and light boxes are connected by a sliding door. The process of learning and preliminary training of rats is done in 4 days. For this purpose, on the first and second days, the rat becomes familiar with the box and its parts and is allowed to move freely in the device for 5 min. On the third day, after placing the rat in the light area, it automatically goes to the dark area, then the sliding door is closed, and an electric shock of 5 mA is delivered for 3 sec to the rat by stainless steel rods. On the fourth day, the recall phase is performed and the rat is allowed to stay in the light area for 300 sec and not enter the dark area. A longer latency in the initial entry into the dark part, the total time spent in the light part, and a shorter total time spent in the dark part indicate better learning and memory [29].
Elevated Plus Maze
The elevated plus maze behavioral test is used to measure the level of anxiety in rats. This maze is made of wood and has four arms in the shape of a plus sign. The two front arms have side protection (enclosed arms) and the other two arms lack side protection (open arms). There is also a central area of 10 × 10 cm. The maze is located by means of bases at a height of 50 cm from the ground. The approach to working with the maze involved placing each rat in the central area 14 days post-surgery. Their behaviors during the 5-minute test were filmed and analyzed to determine the following metrics: percentage of time spent in the open arm (OAT%), percentage of open arm entry (OAE%), and the total entries into both open and closed arms, serving as indices of movement activity [30, 31].


Statistical analysis
One-way ANOVA was used for statistical analysis and comparison between experimental groups, and in case of significance, the post hoc Tukey test was employed for pairwise comparisons within groups. All calculations were performed in R software (version R 4.3.0), and p-values of less than 0.05 were considered significant. The graphs were drawn using Graph Pad Prism software (version 9.4.0.673). The results were shown as SEM ± Mean.
Results
Effects of urolithin A and B in passive avoidance learning test
Figures 1 and 2 display the latency to enter the dark box and the duration of staying there in different groups, respectively. Compared to the control group, the latency to enter the dark box in the negative control group (experimental Alzheimer’s disease) was significantly shorter (P<0.001), while the duration of staying there was significantly longer (P<0.001), showing memory and learning disorders due to intraventricular-cerebral injection of streptozotocin. Although the administration of memantine in the positive control group and drug treatment with urolithin A and B in the experimental groups could improve the time variables, they had no significant differences with the control group.
In the urolithin A groups (20 and 40 mg/kg), in comparison to the negative control group, the latency to enter the dark box was significantly longer (P<0.001 and P<0.05, respectively) and the duration of staying there was also significantly shorter (P<0.001 and P<0.01, respectively). In the urolithin B groups (10 and 20 mg/kg), compared to the negative control group, the latency to enter the dark box was significantly longer (P<0.01), whereas the length of staying there was significantly shorter (P<0.001 and P<0.01, respectively). Although urolithin A (10 mg/kg) and urolithin B (5 mg/kg) could improve the latency to enter the dark box and the length of stay there, they showed no significant differences with the negative control group. Moreover, no significant difference was observed between the control and sham groups
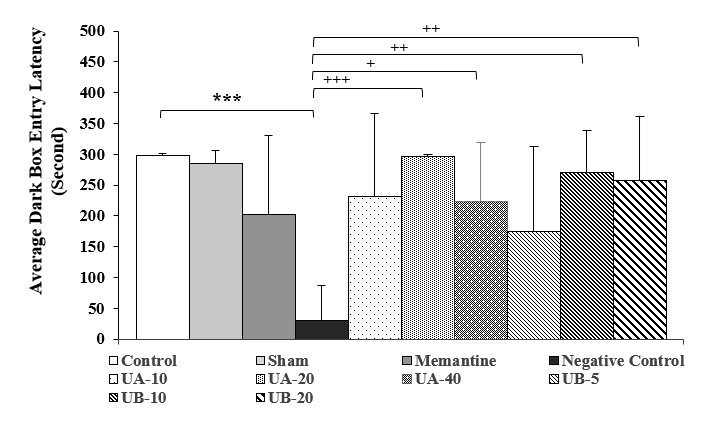

![]()
Figure 1. Comparison of mean latency to enter the dark box in the second week after streptozotocin injection. Results are shown as mean
± SEM (n=7). ***(P<0.001) compared to the control group, +(P<0.05), ++(P<0.01), and +++(P<0.001) compared to the negative control group.
.png)

![]()
Figure 2. Comparison of mean time spent in the dark box during the second week after streptozotocin injection. Results are shown as mean ± SEM (n=7). ***(P<0.001) compared to the control group, ++(P<0.01) and +++(P<0.001) compared to the negative control group.
Effects of urolithin A and B in elevated plus maze test
According to the results of Figure 3, the OAT% in the negative control group decreased significantly (P<0.001), compared to the control group. Administration of memantine in the positive control group, compared to the negative control group, significantly increased the OAT% (P<0.05). In the groups treated with urolithin A (10, 20, and 40 mg/kg), there was a significant increase (P<0.001, P<0.001, and P<0.01, respectively) compared to the negative control group. The OAT% in the groups treated with urolithin B (10 and 20 mg/kg), increased significantly (P<0.001), compared to the negative control group, which showed that urolithin B performed better than memantine as a standard drug. No significant difference was observed between the control and sham groups.
The results of Figure 4 illustrate that the OAE% in the negative control group (experimental Alzheimer’s disease) decreased significantly (P<0.001), compared to the control group. However, a significant increase was observed in the groups treated with urolithin A (10, 20, and 40 mg/kg) and urolithin B (10 and 20 mg/kg), compared to the negative control group (P<0.001). The OAE% increased in the positive control (memantine) and urolithin B (5 mg/kg) groups; nevertheless, it was not statistically significant.
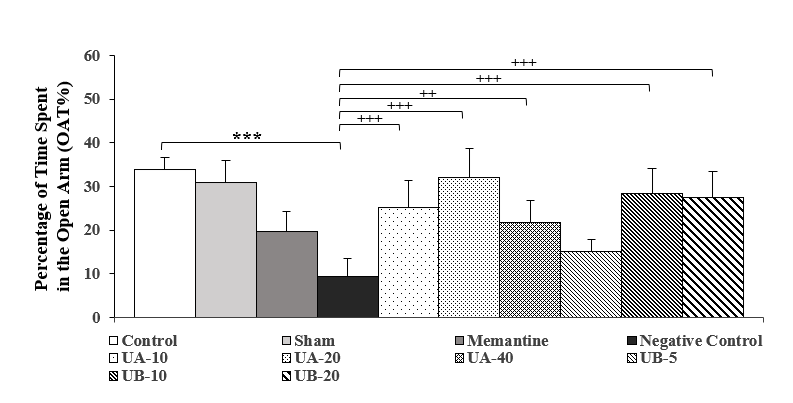

![]()
Figure 3. Comparison of the percentage of time spent in the open arm in the second week after streptozotocin injection. Results are shown as mean ± SEM (n=7). ***(P<0.001) compared to the control group, ++(P<0.01) and +++(P<0.001) compared to the negative control group.


![]()
Figure 4. Comparison of the percentage of the number of entries into the open arm in the second week after streptozotocin injection. Results are shown as mean ± SEM (n=7). ***(P<0.001) compared to the control group, +++(P<0.001) compared to the negative control group.
According to Figure 5, the movement activity in the negative control group decreased, compared to the control group; however, it was not statistically significant. Moreover, in the groups treated with memantine and urolithin A and B, this index increased relatively, in comparison to the negative control group, which was not statistically significant.
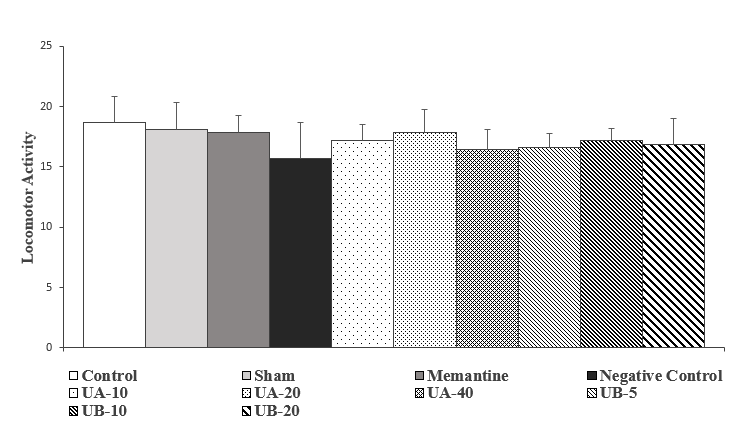

![]()
Figure 5. Comparison of motor activity in the second week after streptozotocin injection. Results are shown as mean ± SEM (n=7). There is no statistically significant difference between the groups.
Discussion
Alzheimer’s disease is a progressive and irreversible neurological disorder characterized by cognitive symptoms (e.g., memory impairment) [32] and non-cognitive symptoms (anxiety) [33]. Currently, there is no effective treatment for Alzheimer’s disease, and many researchers around the world are conducting research and investigation on patients or laboratory animal samples in order to obtain the appropriate medication for the treatment of disorders caused by this disease [34]. Memantine is a drug used to treat the symptoms of Alzheimer’s disease and is a noncompetitive antagonist with a moderate affinity for NMDA glutamate receptors. This drug is used in the treatment of moderate to severe Alzheimer’s disease and is presently the only approved option for this purpose. Memantine improves the ability to think and remember and slows down the process of losing these abilities in people with Alzheimer’s [35].
The results of a study in 2006 showed that the administration of memantine to adult male rats led to a dose-dependent increase in their long-term memory [36]. The results of another study in 2010, which included male and female participants under the age of 50, revealed that treatment with memantine (20 mg per day) improved daily functions in patients with moderate to severe Alzheimer’s disease [37].
One of the laboratory methods for inducing Alzheimer’s disease in animal samples is the intraventricular-cerebral injection of streptozotocin. In this regard, it has been reported that Wistar rats exposed to intraventricular-cerebral injection of streptozotocin suffer from learning and memory impairments, cognitive disorders, and anxiety [33, 38]. Another study was dedicated to investigating the rate of learning and spatial memory of rats by Morris water maze 14 days after the intraventricularcerebral injection of 2, 3, 4, and 5 mg/kg of streptozotocin. The results of this study demonstrated that the injection of 3 mg/kg of streptozotocin was the effective and the baseline dose for causing memory and learning impairments in the shortest possible time (14 days) for the development of early symptoms of Alzheimer’s disease, including the appearance of beta-amyloid plaques in the hippocampus and the increase in the production of reactive species in the mitochondria of the rats' brain [39]. Streptozotocin in the brain causes dysfunction of cholinergic signaling, beta-amyloid accumulation, and decreased insulin receptor sensitivity [40].
The findings of a study showed that one week after the intracerebroventricular injection of streptozotocin, active microglia and astrocytes were detected in the cerebral cortex and around the cannulation site, in the hippocampus, corpus callosum, and medial and lateral septum, and 3 weeks later from the injection of streptozotocin, memory impairment was confirmed in rats [41]. From a cognitive and non-cognitive symptoms perspective, the results of the present study demonstrated that intraventricular-cerebral injection of streptozotocin to male rats induced an experimental model of Alzheimer’s disease, and treatment with urolithin was able to significantly halt the progression of the disease or improve disease symptoms (Figures 1 and 2).
In the behavioral test of passive avoidance learning, the latency in entering the dark box and the increase in time spent there (learning or memory impairment) in the negative control group indicated the negative effect of intraventricular injection of streptozotocin in the process of learning and memory (induction of experimental Alzheimer’s). On the other hand, an increase in the latency in entering the dark box in the experimental groups showed the positive effects of urolithin in improving the learning process or the memory recall process.
The results of examining the oral administration of ellagic acid to diabetic rats induced by intraperitoneal injection of streptozotocin for eight weeks demonstrated that ellagic acid reduced anxiety-like behaviors and depression symptoms and improved exploratory/motor activities and cognitive defects in diabetic rats. These results were associated with the reduction of blood glucose levels, modulation of inflammation, and improvement of neuronal destruction in diabetic rats, and also showed that in some aspects, treatment with ellagic acid was even more effective than insulin [42].
The results obtained from the elevated plus maze test were also in line with those obtained from the shuttle box test (Figures 3 and 4). In this regard, a decrease in the OAT% and OAE% in the negative control group, compared to the control group, indicated anxiety-like behaviors, while an increase of these variables in the experimental groups showed the positive effects of urolithin administration in reducing anxiety-like behaviors in these groups. Published reports demonstrate that the occurrence of anxiety-like behaviors is one of the characteristics of dementia in different laboratory models [43]. In rats, intraventricular-cerebral injection of streptozotocin causes severe disorders in the downstream metabolic pathways of the insulin signaling cascade [44, 45] through the reduction of insulin receptor sensitivity and the activity of glycolytic enzymes, followed by the occurrence of memory and learning impairments and increased anxiety [46].
To stop or slow down the progress of Alzheimer’s disease, in addition to drug therapy, various strategies, such as gene therapy, have also been suggested [47]. At the same time, the identification of bioactive substances and alternative natural products with the aim of discovering potential candidates with antioxidant and anti-inflammatory properties is still on the agenda of researchers in this field [48]. According to published reports, some natural bioactive compounds, including flavonoids, improve brain function and memory [49]. Urolitins (urolithins A and B) are among the flavonoids that are derived from the metabolism of ellagic acid by the gut microbiota at the end of the gastrointestinal tract. Owing to their antioxidant and anti-inflammatory properties [12], these substances have probably positive effects on brain disorders, especially memory. These compounds have a higher absorption rate and better biocompatibility because of their specific biochemical structure [23].
The results of a study showed that ellagic acid can reduce the severity of neurological disorders caused by ischemic hypoperfusion due to its antioxidant properties and free radical inhibition [50]. The findings of examining and investigating the effect of urolithin A on a transgenic mouse model of Alzheimer’s disease demonstrated that this substance reduced the accumulation and deposition of amyloid beta [51]. The results of a study in 2021 showed that urolithin A had neuroprotective activity, and treatment with this substance in a cell model of early Alzheimer’s disease caused the transcription of several genes related to mitochondrial biogenesis and reduced its dysfunction [52]. The study and investigation of urolithin A injection in a streptozotocin-induced diabetic mouse model in 2021 showed that urolithin A reduced the expression of amyloid beta enzymes and the formation of amyloid plaque [53].
The results of another study in 2023 showed that urolithin A in a transgenic mouse model of Alzheimer’s disease prevented spatial memory and exploratory behavior disorders and also reduced beta-amyloid plaques in the hippocampus [54]. The study and examination of human nerve cells in the culture medium treated with urolithin B showed that this substance led to significant inhibition of the formation of the final product of glycosylation and a reduction of mild oxidative stress in an ex vivo experimental model [55]. A study was conducted in 2020 to investigate the activity of monoamine oxidase type A and B enzymes in the culture medium in the presence or absence of urolithin A, B, C, and ellagic acid. The results of this study demonstrated that urolithin B was a strong inhibitor of monoamine oxidase type A, and it could have beneficial effects in reducing depression symptoms because urolithins can pass the blood-brain barrier [56].
The results of the present behavioral study showed that intraperitoneal injection of urolithin A and B could improve learning and memory and reduce anxiety-like behaviors in the Alzheimer’s rat model. In line with these findings, in a report published in 2019, it has been shown that the oral consumption of urolithin A led to a decrease in the accumulation of amyloid plaques in the cerebral cortex and hippocampus of Alzheimer’s mouse model, as well as a decrease in apoptosis in D-galactose-induced brain aging in mice [57].
In addition, the results of a study in 2021 showed that pretreatment with urolithin B in a mouse model of D-galactose-induced age-related neurodegenerative disease increased the survival of neurons by protecting against oxidative stress, and ultimately, led to improvement in neurological deficits and cognitive function. This study suggested urolithin B as an effective supplement to prevent memory impairment and brain damage caused by oxidative stress [58]. Moreover, the results of in vitro experiments have shown that the addition of urolithin A and B to the culture medium of microglia cells decreased nitric oxide production and mRNA levels of pro-inflammatory cytokines, such as TNF-α and IL-6 [59].
Conclusion
The results of this study showed that intraperitoneal administration of urolithins A and B had positive effects on learning and memory as well as anxiety-like behaviors in the rat model of Alzheimer’s disease induced by streptozotocin. It seems that urolithins A and B have the potential to be considered one of the promising therapeutic candidates for halting or preventing the progression of neurodegenerative diseases.
Recommendations
According to the results obtained from the behavioral tests conducted in this research, it is recommended to conduct further study to investigate the effects of urolithin A and B on the gene
expression levels of TNFα and NRF2 factors, acetylcholinesterase and nitric oxide activity, as well as inflammatory factors in the brain tissue of the mouse model of Alzheimer’s disease.
Ethical Considerations
Compliance with ethical guidelines
This study was approved by the Ethics Committee of Ferdowsi University of Mashhad under the ethics code IR.UM.REC.1402.058 in letter no. 3/60290 dated 03.07.2023 in the meeting held on 09-02.
Funding
This study was a part of the master's thesis in the field of animal physiology and was carried out with the financial support of Ferdowsi University of Mashhad.
Authors' contributions
All authors contributed equally to this article.
Conflicts of interest
According to the authors, there is no conflict of interest in publishing this article.
Acknowledgments
The authors would like to express their gratitude to the Department of Pharmacognosy, Faculty of Pharmacy, Mashhad University of Medical Sciences, for their cooperation and assistance in conducting this study.
References
- Wan YW, Al-Ouran R, Mangleburg CG, Perumal TM, Lee TV, Allison K, et al. Meta-analysis of the Alzheimer's disease human brain transcriptome and functional dissection in mouse models. Cell Rep. 2020;32(2):107908. [DOI: 10.1016/j.celrep.2020.107908] [PMID] [PMCID]
- Hebert LE, Scherr PA, Beckett LA, Albert MS, Pilgrim DM, Chown MJ, Funkenstein HH, Evans DA. Age-specific incidence of Alzheimer's disease in a community population. JAMA. 1995;273(17):1354-9. [PMID]
- Tahami Monfared AA, Byrnes MJ, White LA, Zhang Q. Alzheimer's Disease: Epidemiology and Clinical Progression. Neurol Ther. 2022;11(2):553-69. [DOI: 10.1007/s40120-022-00338-8] [PMID] [PMCID]
- Breijyeh Z, Karaman R. Comprehensive Review on Alzheimer's Disease: Causes and Treatment. Molecules. 2020;25(24):5789. [DOI: 10.3390/molecules25245789] [PMID] [PMCID]
- Vaz M, Silvestre S. Alzheimer's disease: Recent treatment strategies. Eur J Pharmacol. 2020;887:173554. [DOI: 10.1016/j.ejphar.2020.173554] [PMID]
- Yiannopoulou KG, Papageorgiou SG. Current and Future Treatments in Alzheimer Disease: An Update. J Cent Nerv Syst Dis. 2020;12:1179573520907397. [DOI: 10.1177/1179573520907397] [PMID] [PMCID]
- Sperling RA, Dickerson BC, Pihlajamaki M, Vannini P, LaViolette PS, Vitolo OV, et al. Functional alterations in memory networks in early Alzheimer's disease. Neuromolecular Med. 2010;12(1):27-43. [DOI: 10.1007/s12017-009-8109-7] [PMID] [PMCID]
- Jahn H. Memory loss in Alzheimer's disease.
Dialogues Clin Neurosci. 2013;15(4):445-54. [DOI: 10.31887/DCNS.2013.15.4/hjahn] [PMID] [PMCID]
- Grossberg GT. The ABC of Alzheimer's disease: behavioral symptoms and their treatment. Int Psychogeriatr. 2002;14(1):27-49. [DOI: 10.1017/s1041610203008652] [PMID]
- Mendez MF. The Relationship Between Anxiety and Alzheimer's Disease. J Alzheimers Dis Rep. 2021;5(1):171-7. [DOI: 10.3233/ADR-210294] [PMID]
- Tendero C, Tixier C, Tristant P, Desmaison J, Leprince P.. Atmospheric pressure plasmas: A review. Spectrochimica Acta Part B: Atomic Spectroscopy. 2006;61(1):2-30. [DOI: 10.1016/j.sab.2005.10.003]
- Hasheminezhad SH, Boozari M, Iranshahi M, Yazarlu O, Sahebkar A, Hasanpour M, Iranshahy M. A mechanistic insight into the biological activities of urolithins as gut microbial metabolites of ellagitannins. Phytother Res. 2022;36(1):112-46. [DOI: 10.1002/ptr.7290] [PMID]
- Förstl H, Kurz A. Clinical features of Alzheimer's disease. Eur Arch Psychiatry Clin Neurosci. 1999;249(6):288-90. [DOI: 10.1007/s004060050101] [PMID]
- Kamat PK, Kalani A, Tyagi SC, Tyagi N. Hydrogen Sulfide Epigenetically Attenuates Homocysteine-Induced Mitochondrial Toxicity Mediated Through NMDA Receptor in Mouse Brain Endothelial (bEnd3) Cells. J Cell Physiol. 2015;230(2):378-94. [DOI: 10.1002/jcp.24722] [PMID]
- Qing-Qing Xu, Wen Yang and Mei Zhong et al. Animal models of Alzheimer’s disease: preclinical insights and challenges. Acta Materia Medica. 2023;2(2):192-215. [DOI: 10.15212/AMM-2023-0001]
- Costa DC, Costa HS, Albuquerque TG, Ramos F, Castilho MC, Sanches-Silva A. Advances in phenolic compounds analysis of aromatic plants and their potential applications. Trends
in Food Science & Technology. 2015;45(2):336-54. [DOI: 10.1016%2Fj.tifs.2015.06.009]
- Pavlica S, Gebhardt R. Protective effects of flavonoids and two metabolites against oxidative stress in neuronal PC12 cells. Life Sci. 2010;86(3-4):79-86. [DOI: 10.1016/j.lfs.2009.10.017] [PMID]
- Orhan DD, Ozçelik B, Ozgen S, Ergun F. Antibacterial, antifungal, and antiviral activities of some flavonoids. Microbiol Res. 2010;165(6):496-504. [DOI: 10.1016/j.micres.2009.09.002] [PMID]
- Hwang SL, Shih PH, Yen GC. Neuroprotective effects of citrus flavonoids. J Agric Food Chem. 2012;60(4):877-85. [DOI: 10.1021/jf204452y] [PMID]
- Landete JM. Ellagitannins, ellagic acid and their derived metabolites: A review about source, metabolism, functions and health. Food research international. 2011;44(5):1150-60. [DOI: 10.1016/j.foodres.2011.04.027]
- A Marshall D, J Stringer S, D Spiers J. Stilbene, ellagic acid, flavonol, and phenolic content of muscadine grape (Vitis rotundifolia Michx.) cultivars. Pharmaceutical Crops. 2012;3(1). [DOI: 10.2174/2210290601203010069]
- Heber D, Seeram NP, Wyatt H, Henning SM, Zhang Y, Ogden LG, Dreher M, Hill JO. Safety and antioxidant activity of a pomegranate ellagitannin-enriched polyphenol dietary supplement in overweight individuals with increased waist size. J Agric Food Chem. 2007;55(24):10050-4. [DOI: 10.1021/jf071689v] [PMID]
- Cerdá B, Espín JC, Parra S, Martínez P, Tomás-Barberán FA. The potent in vitro antioxidant ellagitannins from pomegranate juice are metabolised into bioavailable but poor antioxidant hydroxy-6H-dibenzopyran-6-one derivatives by the colonic microflora of healthy humans. Eur J Nutr. 2004;43(4):205-20. [DOI: 10.1007/s00394-004-0461-7] [PMID]
- Bialonska D, Ramnani P, Kasimsetty SG, Muntha KR, Gibson GR, Ferreira D. The influence of pomegranate by-product and punicalagins on selected groups of human intestinal microbiota. Int J Food Microbiol. 2010;140(2-3):175-82. [DOI: 10.1016/j.ijfoodmicro.2010.03.038] [PMID]
- García-Villalba R, Selma MV, Espín JC, Tomás-Barberán FA. Identification of Novel Urolithin Metabolites in Human Feces and Urine after the Intake of a Pomegranate Extract. J Agric Food Chem. 2019;67(40):11099-107. [DOI: 10.1021/acs.jafc.9b04435] [PMID]
- Zhang M, Cui S, Mao B, Zhang Q, Zhao J, Zhang H, Tang X, Chen W. Ellagic acid and intestinal microflora metabolite urolithin A: A review on its sources, metabolic distribution, health benefits, and biotransformation. Crit Rev Food Sci Nutr. 2023;63(24):6900-22. [DOI: 10.1080/10408398.2022.2036693] [PMID]
- Tsukano H, Horie M, Hishida R, Takahashi K, Takebayashi H, Shibuki K. Quantitative map of multiple auditory cortical regions with a stereotaxic fine-scale atlas of the mouse brain. Sci Rep. 2016;6:22315. [DOI: 10.1038/srep22315] [PMID] [PMCID]
- Paxinos G, Watson C, Pennisi M, Topple A. Bregma, lambda and the interaural midpoint in stereotaxic surgery with rats of different sex, strain and weight. J Neurosci Methods. 1985;13(2):139-43. [DOI: 10.1016/0165-0270(85)90026-3] [PMID]
- Schliebs R, Arendt T. The cholinergic system in aging and neuronal degeneration. Behav Brain Res. 2011;221(2):555-63. [DOI: 10.1016/j.bbr.2010.11.058] [PMID]
- Rodgers RJ, Johnson NJ. Factor analysis of spatiotemporal and ethological measures in the murine elevated plus-maze test of anxiety. Pharmacol Biochem Behav. 1995;52(2):297-303. [DOI: 10.1016/0091-3057(95)00138-m] [PMID]
- Rezayat M, Roohbakhsh A, Zarrindast MR, Massoudi R, Djahanguiri B. Cholecystokinin and GABA interaction in the dorsal hippocampus of rats in the elevated plus-maze test of anxiety. Physiol Behav. 2005;84(5):775-82. [DOI: 10.1016/j.physbeh.2005.03.002] [PMID]
- Woodward M. Aspects of communication in Alzheimer's disease: clinical features and treatment options. Int Psychogeriatr. 2013;25(6):877-85. [DOI: 10.1017/S1041610213000318] [PMID]
- Gao C, Liu Y, Jiang Y, Ding J, Li L. Geniposide ameliorates learning memory deficits, reduces tau phosphorylation and decreases apoptosis via GSK3β pathway in streptozotocin-induced alzheimer rat model. Brain Pathol. 2014;24(3):261-9. [DOI: 10.1111/bpa.12116] [PMID]
- Dubey SK, Parab S, Alexander A, Agrawal M, Achalla VP, Pal UN, Pandey MM, Kesharwani P. Cold atmospheric plasma therapy in wound healing. Process Biochemistry. 2022;112:112-23. [DOI: 10.1016/j.procbio.2021.11.017]
- McShane R, Westby MJ, Roberts E, Minakaran N, Schneider L, Farrimond LE, Maayan N, Ware J, Debarros J. Memantine for dementia. Cochrane Database Syst Rev. 2019;3(3):CD003154. [DOI: 10.1002/14651858.CD003154.pub6] [PMID] [PMCID]
- Zoladz PR, Campbell AM, Park CR, Schaefer D, Danysz W, Diamond DM. Enhancement of long-term spatial memory in adult rats by the noncompetitive NMDA receptor antagonists, memantine and neramexane. Pharmacol Biochem Behav. 2006;85(2):298-306. [DOI: 10.1016/j.pbb.2006.08.011] [PMID]
- Winblad B, Gauthier S, Aström D, Stender K. Memantine benefits functional abilities in moderate to severe Alzheimer's disease. J Nutr Health Aging. 2010;14(9):770-4. [DOI: 10.1007/s12603-010-0122-x] [PMID]
- Mehla J, Pahuja M, Gupta YK. Streptozotocin-induced sporadic Alzheimer's disease: selection of appropriate dose. J Alzheimers Dis. 2013;33(1):17-21. [DOI: 10.3233/JAD-2012-120958] [PMID]
- Anjomani M, Eliassi A, Ghasemi R, Fahanik-Babaei J. Effect of intracerebroventricular injection of streptozotocin on memory, reactive oxygen species of brain mitochondria, and β-amyloid plaques in rat hippocampus. Iranian Journal of Physiology and Pharmacology. 2020;4(3):130-18. [Link]
- Gutierres JM, Carvalho FB, Schetinger MR, Marisco P, Agostinho P, Rodrigues M. Anthocyanins restore behavioral and biochemical changes caused by streptozotocin-induced sporadic dementia of Alzheimer's type. Life Sci. 2014;96(1-2):7-17. [DOI: 10.1016/j.lfs.2013.11.014] [PMID]
- Shoham S, Bejar C, Kovalev E, Schorer-Apelbaum D, Weinstock M. Ladostigil prevents gliosis, oxidative-nitrative stress and memory deficits induced by intracerebroventricular injection of streptozotocin in rats. Neuropharmacology. 2007;52(3):836-43. [DOI: 10.1016/j.neuropharm.2006.10.005] [PMID]
- Farbood Y, Rashno M, Ghaderi S, Khoshnam SE, Sarkaki A, Rashidi K, Rashno M, Badavi M. Ellagic acid protects against diabetes-associated behavioral deficits in rats: Possible involved mechanisms. Life Sci. 2019;225:8-19. [DOI: 10.1016/j.lfs.2019.03.078] [PMID]
- Pamplona FA, Pandolfo P, Duarte FS, Takahashi RN, Prediger RD. Altered emotionality leads to increased pain tolerance in amyloid beta (Abeta1-40) peptide-treated mice. Behav Brain Res. 2010;212(1):96-102. [DOI: 10.1016/j.bbr.2010.03.052] [PMID]
- Grünblatt E, Salkovic-Petrisic M, Osmanovic J, Riederer P, Hoyer S. Brain insulin system dysfunction in streptozotocin intracerebroventricularly treated rats generates hyperphosphorylated tau protein. J Neurochem. 2007;101(3):757-70. [DOI: 10.1111/j.1471-4159.2006.04368.x] [PMID]
- Klinkenberg I, Blokland A. The validity of scopolamine as a pharmacological model for cognitive impairment: a review of animal behavioral studies. Neurosci Biobehav Rev. 2010;34(8):1307-50. [DOI: 10.1016/j.neubiorev.2010.04.001] [PMID]
- Marks DR, Tucker K, Cavallin MA, Mast TG, Fadool DA. Awake intranasal insulin delivery modifies protein complexes and alters memory, anxiety, and olfactory behaviors. J Neurosci. 2009;29(20):6734-51. [DOI: 10.1523/JNEUROSCI.1350-09.2009] [PMID] [PMCID]
- Weerateerangkull P, Praputpittaya C, Banjerdpongchai R. Effects of ascorbic acid on streptozotocin-induced oxidative stress and memory impairment in rats. Thai journal of physiological sciences. 2008;20(2):54-68. [Link]
- Cummings JL. Biomarkers in Alzheimer's disease drug development. Alzheimers Dement. 2011;7(3):e13-44. [DOI: 10.1016/j.jalz.2010.06.004] [PMID]
- Carreiras MC, Mendes E, Perry MJ, Francisco AP, Marco-Contelles J. The multifactorial nature of Alzheimer's disease for developing potential therapeutics. Curr Top Med Chem. 2013;13(15):1745-70. [DOI: 10.2174/15680266113139990135] [PMID]
- Ghasemzadeh DM, Rafieirad M, Rouhi L. The Effect of Ellagic Acid on Memory and Pain Induced by Brain Ischemia in Adult Male Rats. Journal of Zanjan University of Medical Sciences & Health Services. 2014;22(92):33-42. [Link]
- Gong Z, Huang J, Xu B, Ou Z, Zhang L, Lin X. Urolithin A attenuates memory impairment and neuroinflammation in APP/PS1 mice. J Neuroinflammation. 2019;16(1):62. [DOI: 10.1186/s12974-019-1450-3] [PMID] [PMCID]
- Esselun C, Theyssen E, Eckert GP. Effects of Urolithin A on Mitochondrial Parameters in a Cellular Model of Early Alzheimer Disease. Int J Mol Sci. 2021;22(15):8333. [DOI: 10.3390/ijms22158333] [PMID] [PMCID]
- Lee HJ, Jung YH, Choi GE, Kim JS, Chae CW, Lim JR, et al. Urolithin A suppresses high glucose-induced neuronal amyloidogenesis by modulating TGM2-dependent ER-mitochondria contacts and calcium homeostasis. Cell Death Differ. 2021;28(1):184-202. [DOI: 10.1038/s41418-020-0593-1] [PMID] [PMCID]
- Ballesteros-Álvarez J, Nguyen W, Sivapatham R, Rane A, Andersen JK. Urolithin A reduces amyloid-beta load and improves cognitive deficits uncorrelated with plaque burden in a mouse model of Alzheimer's disease. Geroscience. 2023;45(2):1095-113. [DOI: 10.1007/s11357-022-00708-y] [PMID]
- Verzelloni E, Pellacani C, Tagliazucchi D, Tagliaferri S, Calani L, Costa LG, et al. Antiglycative and neuroprotective activity of colon‐derived polyphenol catabolites. Molecular Nutrition & Food Research. 2011;55(S1):S35-43. [DOI: 10.1002/mnfr.201000525]
- Singh R, Chandrashekharappa S, Vemula PK, Haribabu B, Jala VR. Microbial Metabolite Urolithin B Inhibits Recombinant Human Monoamine Oxidase A Enzyme. Metabolites. 2020;10(6):258. [DOI: 10.3390/metabo10060258] [PMID] [PMCID]
- Chen P, Chen F, Lei J, Li Q, Zhou B. Activation of the miR-34a-Mediated SIRT1/mTOR Signaling Pathway by Urolithin A Attenuates D-Galactose-Induced Brain Aging in Mice. Neurotherapeutics. 2019;16(4):1269-82. [DOI: 10.1007/s13311-019-00753-0] [PMID] [PMCID]
- Chen P, Chen F, Lei J, Wang G, Zhou B. The Gut Microbiota Metabolite Urolithin B Improves Cognitive Deficits by Inhibiting Cyt C-Mediated Apoptosis and Promoting the Survival of Neurons Through the PI3K Pathway in Aging Mice. Front Pharmacol. 2021;12:768097. [DOI: 10.3389/fphar.2021.768097] [PMID] [PMCID]
- Xu J, Yuan C, Wang G, Luo J, Ma H, Xu L, et al. Urolithins Attenuate LPS-Induced Neuroinflammation in BV2Microglia via MAPK, Akt, and NF-κB Signaling Pathways. J Agric Food Chem. 2018;66(3):571-580. [DOI: 10.1021/acs.jafc.7b03285] [PMID]
پاییز .1401 دوره .28 شماره 4
Type of Study:
Original |
Subject:
Basic Medical Science Received: 2023/08/13 | Accepted: 2024/02/6 | Published: 2023/03/10